Efficiency, efficiency, and efficiency: We are not talking about anything else… it is time to return to the topic of inefficiency, possibly the shortest way to understand the different factors and possible advances in the energy performance of any vehicle.
In today’s chapter we will talk about automobile aerodynamics , but trying to make an understandable approach to such a complex discipline so that no one leaves without being clear about a couple of essential and vitally important things.
Let’s go there?
Aerodynamic drag: a definition
The pressure of the air against the front and the vacuum (suction) generated at the rear combine to generate a force opposing the movement
The first thing to define is the aerodynamic resistance itself , so that we are clear about what we are talking about: it is the force opposite to the movement suffered by a solid object (vehicle) when it moves through the air.
Cars have to remove a significant amount of air to be able to move forward and also make it easier to fill the void they generate behind them . The air pressure against the front and the vacuum (suction) generated at the rear combine to generate a force opposing the movement.
At low speeds, this force is relatively small but, as we will see below, at higher speeds things become more complicated.
At low speeds, this force is relatively small but, as we will see below, at higher speeds things become more complicated . And how.
There is also an upward force , caused by the air that circulates under pressure under the car and by the vacuum generated in the upper rear part, but this vertical force is not such a direct enemy of efficiency (although it generates other problems). so we are not going to include it in the explanation.
Factors that determine aerodynamic drag
Aerodynamic resistance:
R = ½ d x v 2
x A x C x
R = Aerodynamic resistance (N)
d = Air density (kg/m3)
v 2 = velocity squared (m 2 /s 2 )
A = Front surface ( m2)
C x = Aerodynamic drag coefficient
Aerodynamic resistance depends on four factors: the density of the air , the speed squared , the frontal surface and the aerodynamic drag coefficient of the vehicle, all multiplying and therefore influencing to the same extent. If we divide the result of that multiplication by two, we have the complete formula, but what matters here is what each of those factors are and how they act.
The density of the air is approximately constant and we cannot vary it with the design of the vehicle, so I am not going to dedicate any further explanations to it.
From here on, the other three factors of the formula deserve their own section in which to be explained.
Aerodynamic drag depends on speed squared
Speed squared invites us to get into the matter, although it is not a factor that distinguishes one car from another, but only something important that we must understand and that affects everyone equally. Let’s see.
If we multiply the speed by two, the resistance is multiplied by four. Intuitively, someone might think that drag is only linear (double speed would also imply double drag) but it turns out that aerodynamic drag increases much faster than speed
(*) .
(*) The aerodynamic resistance is proportional to the square of the speed because if we double the speed, twice as much air hits the front of the car and it also does so with twice the force: speed x2 implies resistance x4
But the question of speed is even more important: resistance grows with the square of speed, but the power necessary to overcome that resistance grows with the cube of it (!!). This means that when the speed is multiplied by two, the resistance is multiplied by four, and the necessary power by at least eight (**).
(**) The necessary power is proportional to the cube of the speed because if we double the speed the force is multiplied x4 (previous section) and that force is applied for twice the distance per unit of time: speed x2 implies power x8
A numerical example so that more than one can put their hands to their heads: to maintain a constant speed on a horizontal section without wind, any car could need the following powers to overcome its aerodynamic resistance exclusively:
If someone has been surprised by the figures, it is probably because they have understood it correctly.
At 50 km/h around 2 HP
At 100 km/h around 16 HP
At 200 km/h around 128 HP
At 300 km/h around 432 HP
The example is mine, but I don’t think I’m too wrong about an average car (assuming it could reach such speeds). Rolling resistance and mechanical strength would require some additional, much lower power. If anyone is surprised by the figures, it is probably because they understood them correctly.
This is the reason why a small difference in speed (say from 110 km/h to 120 km/h) represents a big difference in consumption. Do you remember that there was a crazy man who was happy that the speed limit was lowered to 110 km/h in Spain? Well, it was me; It’s a shame it lasted so little.
Aerodynamic drag depends on the frontal surface
The front surface is the area occupied by the car seen perfectly from the front. On a plane it would be the elevation of the car, the surface of which depends on the height, width and shape of that elevation (including tires, mirrors and everything that is exposed to the air in the direction of travel).
The interpretation of this is very simple: this front section is the one that sweeps a volume of air per unit of time when the car moves, and defines the mass of air that must be moved away . It is obvious that the more air you move, the more work it will take to do so.
Translated to real cars, cars large in height and width (again, vans, minivans and SUVs) are greatly penalized , but not necessarily long cars, which in fact have more opportunities to make the air flow smoothly around them than the very short ones.
To successfully overcome this obstacle, low and narrow car. The position of tandem passengers in a Renault Twizy , for example, responds in part to this principle, but also to promote city traffic and parking, of course.
Aerodynamic drag depends on the aerodynamic drag coefficient
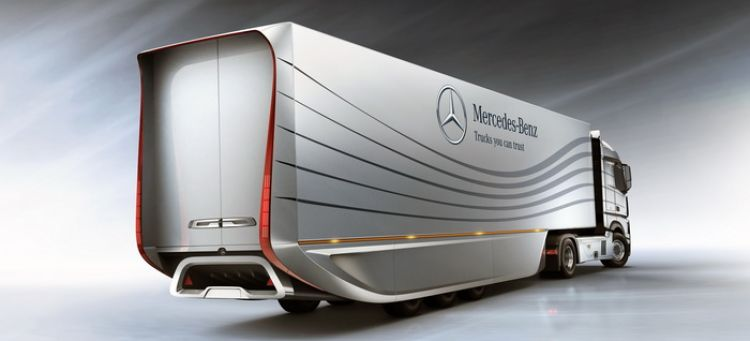
The smoother the curves the airflow has to make around the car, the better.
The aerodynamic drag coefficient (C x ) is a dimensionless number (it has no units) that is determined almost entirely by the shape of the car. The most important thing, contrary to what you might expect, is the rear and the way the air fills the void that the car leaves behind. The smoother the curves the airflow has to take to surround the car, the better.
The interpretation of this factor is easy: the shape of the car defines the path that the air will have to follow to surround it, that is, the speed and arrangement of the air in its different trajectories.
Angular corners, truncated rear corners (vertical and flat as in a van, minivan or SUV) and in general any non-smooth profile that has to travel through the air penalize us in this regard.
It is curious that today it is still not possible to finish developing the aerodynamic aspect of a car without a wind tunnel in which to carry out real tests, but the truth is that computer simulation has not yet reached that level of perfection. , such is the capricious nature of moving air.
With respect to the coefficient itself, it represents the comparison with a square plate with sharp edges whose coefficient C x would be equal to 1. This means that the resistance offered by a vehicle due to its shape with C x = 0.5 would be the half that of the aforementioned rigid plate, and so on.
Conclusions
Aerodynamic drag plays a fundamental role in the efficiency of any vehicle . The power consumption derived from this factor goes from irrelevant to colossal as we increase speed (and power implies fuel consumption).
Vans, minivans and SUVs have a very clear reason for being and a specific utility in exchange for which they are penalized in the aerodynamic section by a large frontal surface and a more difficult way to style.
A more aerodynamic shape (smooth, without edges, with a rounded front and gradually tapering rear) together with contained dimensions in height and width result, other parameters being equal, in more efficient cars.
This is not about banning or demonizing certain types of vehicles. Vans, minivans and SUVs have a very clear reason for being and a specific utility in exchange for which they are penalized in the aerodynamic section by a large frontal surface and a more difficult shape to style. But it is good to know what we are driving and buying, and value it appropriately in all its facets.
Leave a Reply